Introduction
In the quest for sustainable and green energy solutions, solar panels have emerged as a frontrunner, transforming sunlight into electricity that powers our homes, industries, and even cities.
But how do these seemingly simple panels capture the sun’s energy and convert it into electrical power? This article delves into the working principle of solar panels, offering a comprehensive understanding of this clean energy technology.
Section 1: The Basics of Solar Energy Conversion
At the heart of a solar panel’s ability to generate electricity is the photovoltaic (PV) effect. Discovered in 1839 by French physicist Edmond Becquerel, the PV effect is the process by which solar cells within the panel convert sunlight into electricity.
Each solar cell is made primarily of silicon, a semi-conductor material that plays a critical role in this conversion process.
1.1 Structure of a Solar Cell
A solar cell typically consists of two layers of silicon: an n-type silicon layer, which has extra electrons, and a p-type silicon layer, which has extra spaces for electrons called “holes”. When these two layers are placed together, an electric field is created at the junction between them.
Going beyond the basic n-type and p-type silicon layers, modern solar cells incorporate additional layers and materials to enhance performance.
For instance, a passivation layer is often added to the back surface of the cell to reduce recombination of electrons and holes (the places where electrons used to be before being excited by photon energy).
Additionally, anti-reflective coatings are applied to the cell’s surface to minimize the loss of incident light, ensuring that as many photons as possible contribute to the generation of electricity.
Moreover, the introduction of heterojunction technology, which combines amorphous silicon layers with crystalline silicon, has led to solar cells with higher efficiencies. This technology leverages the advantages of both material types to create a more effective electron collection mechanism.
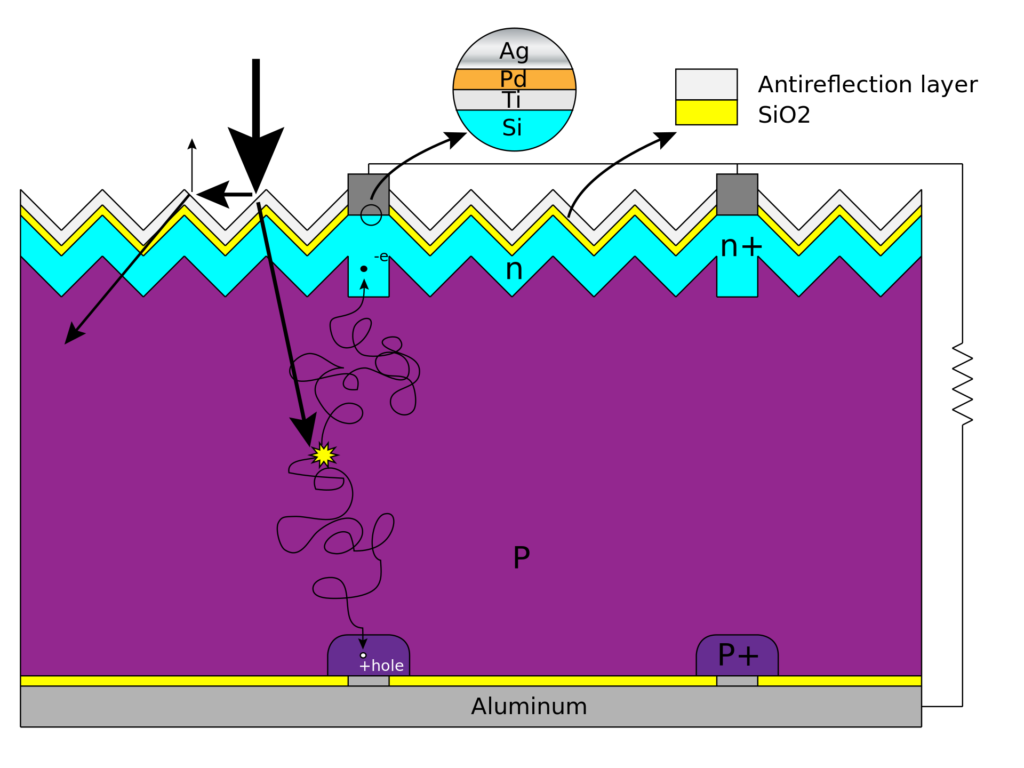
1.2 The Photovoltaic Effect in Action
The photovoltaic (PV) effect is more than just an interaction between sunlight and silicon. It’s a complex interplay of physical and chemical processes that allow for the efficient conversion of light energy into electrical energy.
When photons strike the surface of a solar cell, they don’t just excite electrons; they initiate a cascade of reactions that underscore the beauty of solar energy conversion. This process is highly dependent on the quality of silicon used, as higher purity silicon provides a better medium for the flow of electrons, thereby increasing the efficiency of the solar cell.
The journey of an electron from the moment a photon hits the solar cell to when it generates electrical current involves several steps.
First, the photon’s energy is absorbed by the silicon, creating an electron-hole pair. The built-in electric field at the junction between the n-type and p-type silicon layers then drives these charge carriers in opposite directions, preventing them from recombining.
Electrons move towards the n-type layer, while holes move towards the p-type layer. This movement of charge carriers creates a difference in potential, or voltage, across the cell.
When an external circuit is connected to the solar cell, this voltage drives the flow of electrons through the circuit, delivering power to an external load.
Section 2: From Solar Cells to Solar Panels
While individual solar cells can generate electricity on their own, they are typically assembled together into a solar panel for increased power output. A standard solar panel consists of a series of interconnected solar cells enclosed in a protective glass casing that offers durability and allows sunlight to reach the cells. The back of the panel is a solid backing material, and the entire assembly is framed in metal, providing structure and the ability to mount the panel.
2.1 The Assembly Process
The assembly of solar cells into panels is a precise and careful process that aims to maximize the efficiency and durability of the final product.
Typically, cells are laid out in a grid pattern, allowing for optimal exposure to sunlight. The interconnected cells are then soldered together, a step that requires precision to ensure that the connections are secure and capable of efficiently transferring electric current.
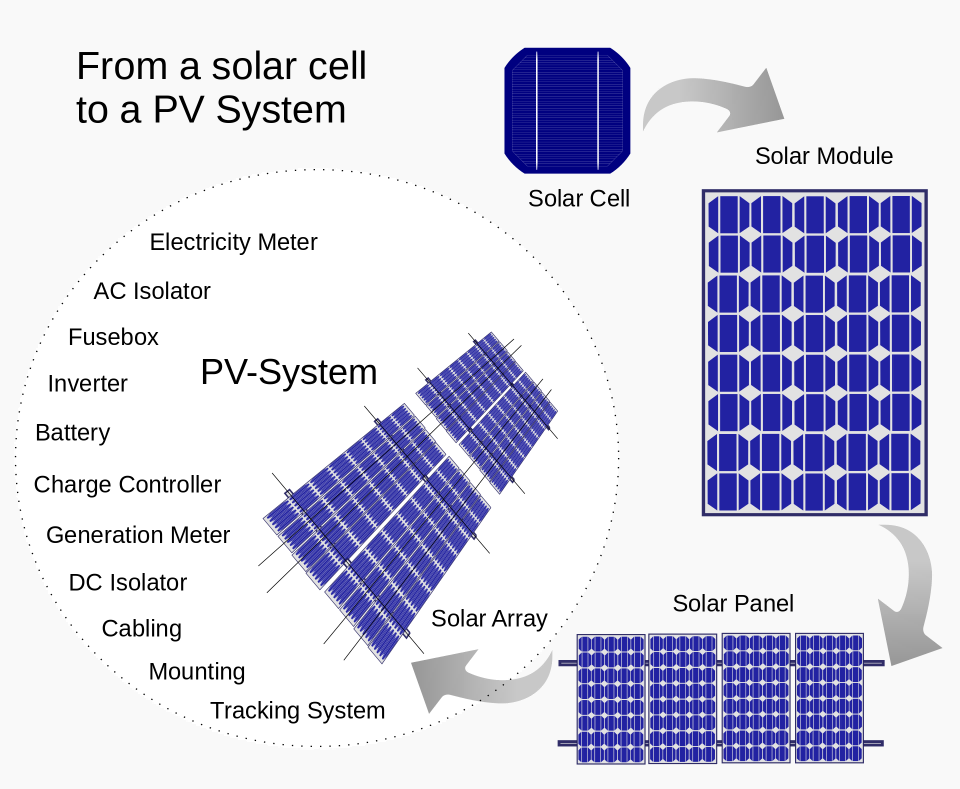
2.2 Encapsulation and Protection
After the cells are interconnected, they are encapsulated in a transparent, protective material, usually EVA (ethylene-vinyl acetate). This layer serves to protect the cells from moisture and physical damage while allowing sunlight to pass through with minimal reflection.
The entire assembly is then placed between a tempered glass front, which offers durability and additional protection against environmental elements, and a back sheet, typically made from a weather-resistant polymer.
This ensures the longevity of the panel by safeguarding against UV damage, moisture ingress, and thermal cycling.
2.3 The Role of the Frame
The metal frame around a solar panel plays a critical role beyond merely providing structure. It ensures the panel’s rigidity and stability, allowing it to withstand high winds, heavy snow loads, and other environmental stresses.
Moreover, the frame facilitates the mounting and grounding of the panel, crucial aspects for both installation and safety purposes.
2.4 Electrical Integration
An often overlooked aspect of solar panels is their electrical integration into a power system. This involves not only the external connections that allow the electricity generated to be used or stored but also the bypass diodes integrated into the panel.
These diodes protect the panel from hot-spot heating, a condition that occurs when a cell is shaded and becomes a significant load rather than a power generator.
2.5 Quality Control and Testing
Before leaving the factory, each solar panel undergoes rigorous testing to ensure it meets specified performance standards.
This includes tests for electrical performance under standard test conditions, durability and reliability testing under accelerated weathering conditions, and mechanical strength testing.
Such stringent quality control measures are essential to guarantee that the panels perform reliably over their intended lifespan, which can span over 25 years.
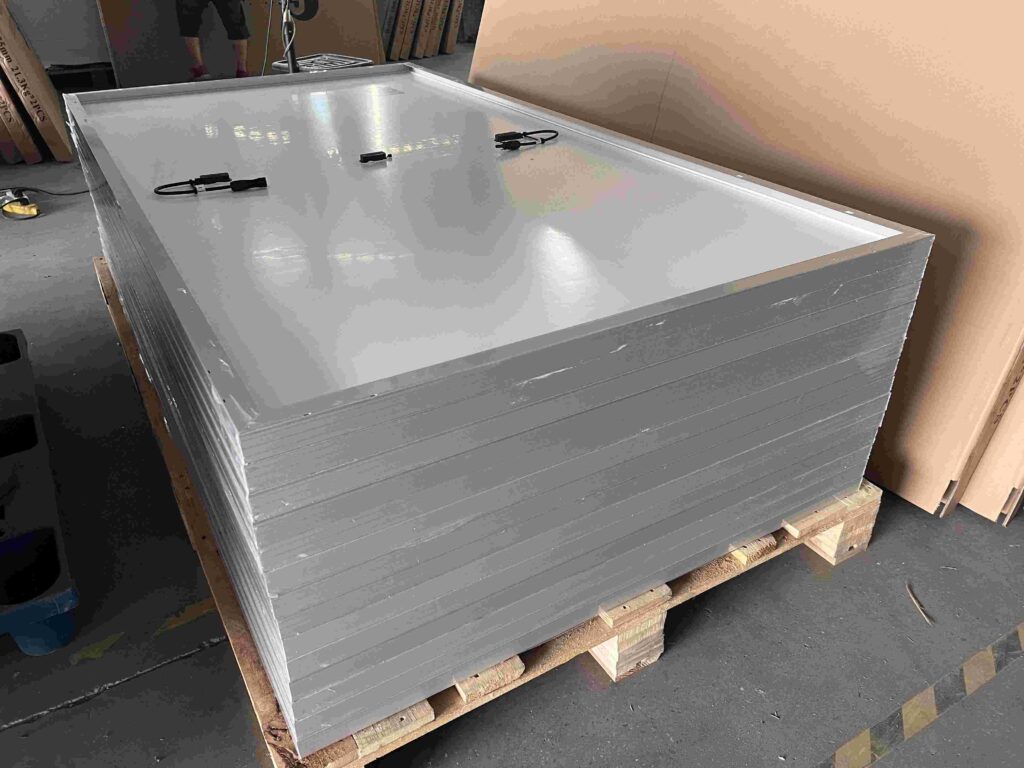
Section 3: Maximizing the Power Output
The efficiency of a solar panel—that is, its ability to convert sunlight into electricity—is determined by several factors, including the quality of the silicon used, the configuration of the solar cells, and the panel’s exposure to sunlight.
3.1 Material Advancements
The silicon used in solar cells is the foundation of their efficiency. Recent developments have led to the creation of more pure forms of silicon, reducing impurities that can impede the flow of electrons.
Furthermore, scientists are exploring alternative materials like perovskite, which offers higher efficiency rates than traditional silicon at potentially lower costs.
These materials are not only more effective at converting sunlight but also open the door to new applications of solar technology, such as flexible and transparent solar panels.
3.2 Cell Design Innovations
The configuration of solar cells within a panel plays a crucial role in maximizing efficiency. Beyond the traditional flat-panel designs, bifacial solar panels are gaining attention. These panels can capture sunlight from both the front and back surfaces, taking advantage of reflective surfaces below the panel.
Additionally, the integration of multi-junction solar cells, which contain layers of different materials each designed to capture a specific segment of the solar spectrum, significantly boosts the overall efficiency.
3.3 Light Absorption Enhancements
The ability of a solar cell to absorb as much light as possible is key to its efficiency. Anti-reflective coatings minimize the loss of sunlight by reducing the reflection off the panel’s surface.
Moreover, textured surfaces can trap more light within the cell, giving photons more opportunities to interact with the silicon.
Manufacturers are also incorporating nanophotonic and plasmonic structures to manipulate light at the nanoscale, enhancing the absorption without increasing the thickness of the solar cell.
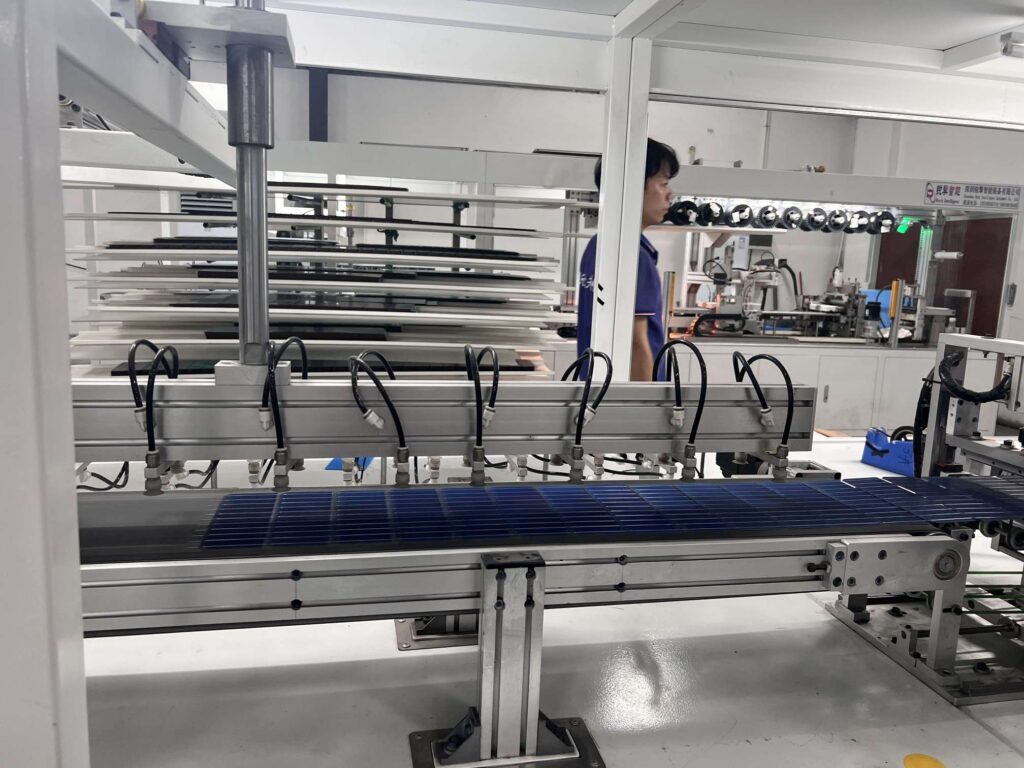
3.4 Concentrated Solar Power (CSP) Technologies
CSP systems use mirrors or lenses to concentrate a large area of sunlight onto a small area of photovoltaic materials. This not only increases the amount of light reaching the cell but also allows for the use of high-efficiency but costly multi-junction solar cells economically.
CSP technologies can include tracking systems that follow the sun’s path across the sky, ensuring that the sunlight remains focused on the photovoltaic material throughout the day.
3.5 Optimizing Panel Placement and Angle
The orientation and angle at which solar panels are installed significantly affect their efficiency. Panels should be positioned to maximize their exposure to direct sunlight throughout the year.
This involves considering the latitude of the installation site and the optimal tilt angle of the panels. Tracking systems that adjust the panel’s angle in response to the sun’s position can further increase the energy production by keeping the panels aligned with the sun throughout the day.
3.6 Addressing Temperature Effects
Solar panel efficiency decreases as temperature increases, a challenge particularly relevant in hot climates.
Advanced cooling technologies, such as passive cooling designs that enhance airflow around the panels or active cooling systems that utilize fluids to dissipate heat, are being developed to mitigate this effect.
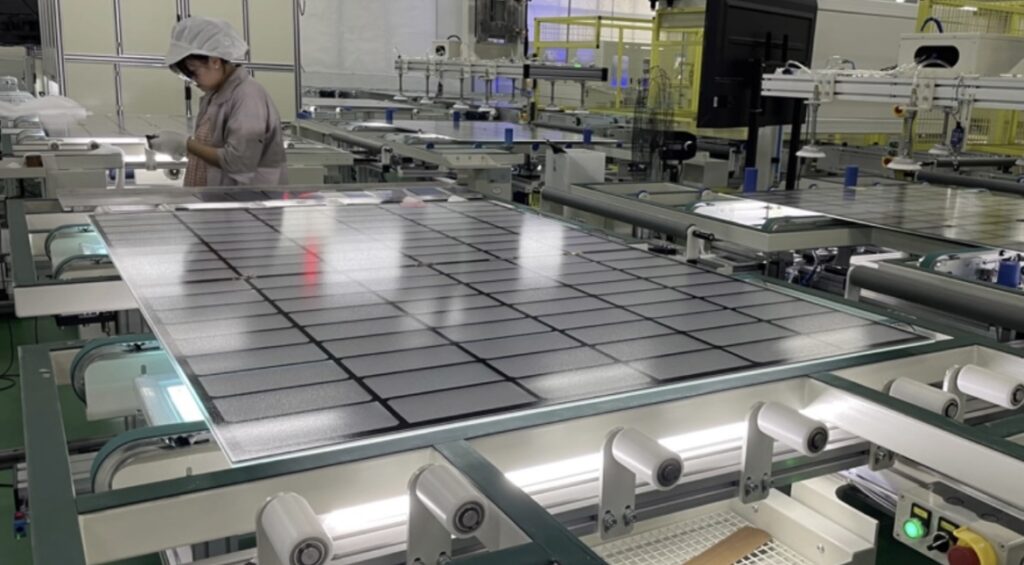
Section 4: Applications and Future Prospects
4.1 Diverse Applications Across Sectors
Solar panels have found their way into a variety of sectors beyond the conventional residential and commercial installations. In agriculture, for instance, solar panels are used to power irrigation systems, reducing the reliance on diesel pumps and grid electricity. This not only lowers operational costs but also mitigates the carbon footprint associated with farming activities.
In remote and rural areas, solar panels provide a lifeline, offering access to electricity where traditional grid infrastructure may be lacking or non-existent. Solar-powered water purification systems bring clean drinking water to communities, while solar lighting extends productive hours after sunset, improving safety and quality of life.
The transportation sector is also embracing solar energy, with innovations like solar-powered electric vehicle (EV) charging stations emerging. These stations are pivotal in addressing the range anxiety associated with EVs, facilitating longer journeys and encouraging the shift away from fossil-fuel-powered vehicles.
4.2 Future Prospects: The Path Forward
The future of solar energy is illuminated by promising technological advancements and an expanding scope of applications. One of the most exciting developments is the advent of floating solar farms. These installations, set on bodies of water, not only generate clean energy but also reduce water evaporation, making them doubly beneficial, especially in arid regions.
Building-integrated photovoltaics (BIPV) are another frontier. BIPVs seamlessly integrate solar cells into building materials, such as windows and facade panels, turning entire structures into energy-generating entities. This approach not only saves space but also enhances the aesthetic appeal of buildings, pushing the boundaries of architectural design.
The integration of energy storage systems with solar panels is set to address one of the main challenges of solar energy: its intermittent nature. Batteries capable of storing solar energy for use during overcast periods or nighttime are becoming more efficient and affordable, paving the way for truly off-grid living and the stabilization of grid energy supplies.
4.3 Innovations in Materials and Efficiency
Research into new photovoltaic materials, like perovskite solar cells, is underway, offering the promise of higher efficiency rates at potentially lower manufacturing costs compared to traditional silicon-based cells. These materials could lead to the production of lightweight, flexible solar panels that could be applied to a wider range of surfaces and objects, further broadening the application of solar technology.
Moreover, the exploration of tandem solar cells, which layer different photovoltaic materials to capture a broader spectrum of sunlight, is poised to break the efficiency limits of current solar panels. By harnessing more of the sun’s energy, these advanced cells could significantly reduce the cost of solar electricity, making it even more competitive with conventional energy sources.

Conclusion
solar panels embody the synergy between nature’s bounty and human innovation, providing a sustainable pathway away from fossil fuels. Through the photovoltaic effect, they convert sunlight into electricity, showcasing solar energy’s vast potential for a cleaner future.
As SolarCtrl continues to lead with over 14 years of expertise in the solar industry, we’re not just witnessing an evolution in renewable energy; we’re actively driving it. Our commitment to pioneering more efficient, accessible solar solutions is unwavering, reflecting our dedication to a sustainable and eco-friendly future.
Embrace the power of the sun with SolarCtrl. Whether you’re looking to make a difference in the world or simply reduce your energy bills, we’re here to help you harness solar energy’s potential. Let’s work together to illuminate a brighter future for our planet. Contact SolarCtrl today, and take the first step towards a sustainable tomorrow.